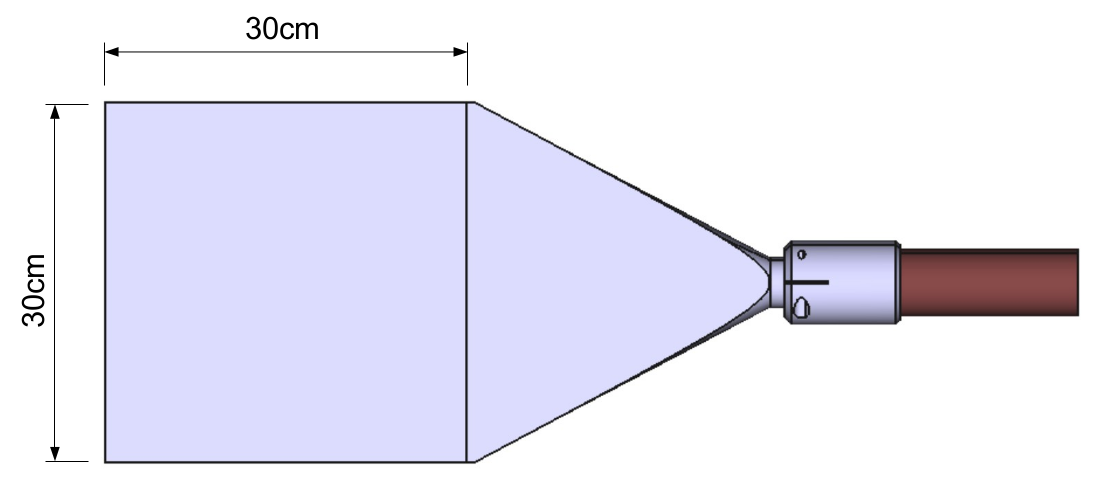
The FASER experiment has four scintillator stations that are used to veto charged particles entering the decay volume from the direction of the ATLAS IP. These are used for both triggering and inducing preshowers.
The first two stations are the veto stations located in front of the dipole magnets and are primarily used to suppress events with charged particles produced externally (i.e. outside the FASER decay volume). These are mostly high-energy muons. The front scintillator stations also effectively suppress backgrounds from any muon-induced particles produced in front of the detector that are associated with a parent muon when entering the FASER detector. To improve rejection of energetic photons from muon bremsstrahlung before the detector, an absorber block of lead is placed between the two veto stations, which either contain the photons completely or generate a shower that is detectable by the second station. An extremely high (>99.99%) vetoing efficiency of charged particles is achieved for each of the stations separately.
Each of the two veto stations consists of two identical scintillator layers. The basic design of each layer is shown in Fig. 1 and consists of a 2 cm-thick, 30 cm × 30 cm plastic scintillator connected through a light guide to a photomultiplier tube (PMT). The PMTs are encased in mu-metal to ensure its operation is not affected by the magnetic fringe field of the FASER magnet. The transverse size of the scintillator layers is larger than the magnet aperture to further ensure no charged particles can enter the decay volume undetected.
The trigger/timing station located after the first magnet and first tracking station is used to detect the presence of a charged particle pair from the decay of an LLP in the decay volume of the first dipole magnet. It provides the primary trigger signal and is also used to precisely measure the arrival time of the signal with respect to the pp interaction at the ATLAS IP. The timing precision is better than 1 ns, which allows suppression of any non-collision backgrounds. The active area of this scintillator station also covers most of front surface of the magnet. This is to detect muons which enter at an angle, miss the veto stations and the first two tracker stations, but cause an electromagnetic shower in the magnet material, thereby inducing a detectable shower in the downstream calorimeter similar to a photon-only signature.
The trigger/timing station design differs from the veto station and is shown in Fig. 2. It consists of a single scintillator layer made from two 20 cm × 40 cm scintillator blocks with a thickness of 1 cm (2.5% of a radiation length). The timing resolution is limited by the precision of the readout electronics.
The final trigger/preshower station is located just in front of the calorimeter. This station provides an additional trigger signal which, if needed, can be used in a coincidence with the first trigger station to reduce the rate of non-physics triggers. It is preceded by a thin layer of radiator (about 1 radiation length of tungsten and graphite) to create a simple preshower detector that with high efficiency can detect a physics signal of two nearby energetic photons. The preshower helps distinguish this physics signal, which would otherwise leave only a large energy deposition in the calorimeter from the deep inelastic scattering of high energy neutrinos. A layer of graphite blocks is installed between the preshower scintillator and calorimeter to reduce backsplash from the calorimeter into the tracker stations
The trigger/preshower station uses two layers of scintillators in the same configuration as the veto stations with half of the radiator material placed in front of the first layer and the second half in between the layers.