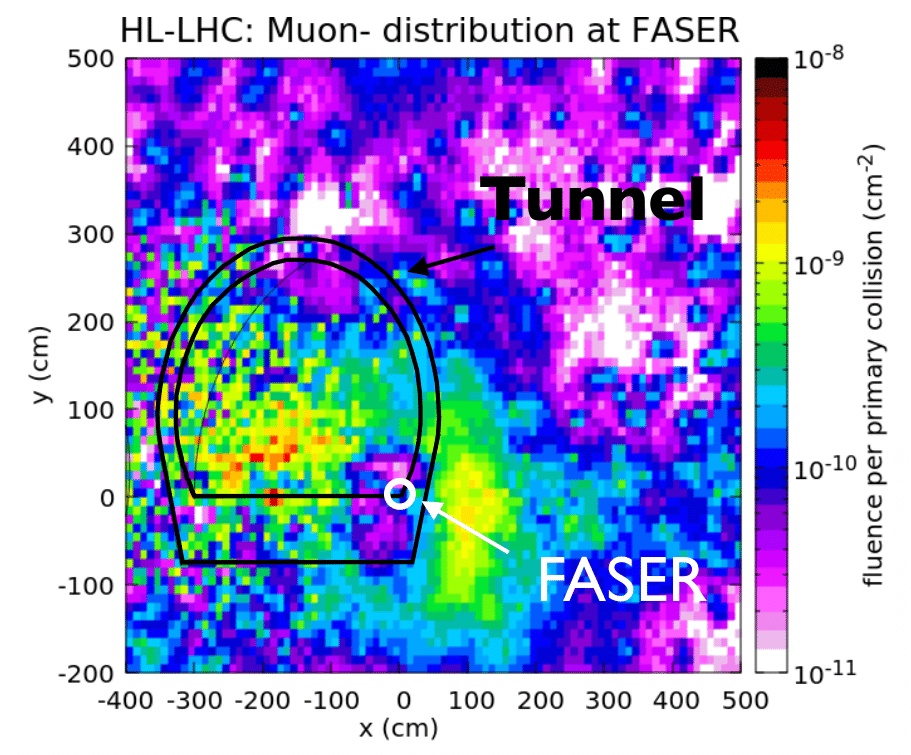
The LHC infrastructure naturally shields the FASER location in tunnel TI12 from most particles produced at the ATLAS IP. The remaining high-energy particles that can reach FASER are muons and neutrinos. Although most of the high-energy muons are deflected by the LHC magnets, some of them can still reach FASER. This is especially true for the ones produced in showers initiated by neutral particles hitting the neutral particle absorber TAN about 140 m away from the ATLAS IP. On the other hand, neutrino interaction cross sections are very low, and, therefore, their scatterings will not contribute to background for searches for light long-lived particles.
Detailed FLUKA simulations of the standard model background at FASER's location have been performed by the CERN EN-STI group. These simulations take into account the impact of the LHC magnets and other elements of the infrastructure. Fig. 1 shows the simulated particle fluence rates (normalized to an instantaneous luminosity of 2 × 10 34 cm−2s−1 and collision energy of 13 TeV) as functions of muon energy. Importantly, the flux of muons through the TI12 tunnel is significantly larger for positions horizontally displaced from the beam collision axis as a result of the impact of the LHC optics. Therefore, the FASER detector is placed in a region with locally reduced particle flux, as illustrated in Fig. 2, where the negative muon flux as a function of radial position around FASER is shown (similar results are obtained for positive muons).
Particles may also be produced in beam-gas collisions as well as proton-loss-induced showers in the dispersion suppressors at the transition between the LHC insertion and arc regions. The CERN EN-STI group performed an accurate modeling of these effects, concluding that beam-related backgrounds are also negligible. In particular, beam-gas interaction rates are extremely small, due to the excellent vacuum in the LHC beam pipe. Furthermore, the dispersion function close to the FASER location minimizes proton losses in this region. Therefore, these backgrounds do not contribute to the high-energy particle flux, and the resulting particles also have the wrong directionality.
On top of simulations, in situ measurements of particle fluxes at FASER's location have also been performed with emulsion detectors installed at the experimental site during LHC Run 2 (see photo in Fig. 3). The angular distribution of particles detected is shown in Fig. 4, which shows a clear peak of charged particles entering the detector from a direction compatible with the ATLAS IP, as the peak population of tracks is close to the origin (0,0) in the figures. The measured particle flux in the main peak (which is within 10 mrad of the beam collision axis) is ~1.9x104 fb cm-2 and agrees well with the results of simulations. A small secondary peak visible in the angular distribution corresponds to low-energy tracks entering the detector that originate from the LHC beamline at the bottom of the TI12 tunnel. These tracks do not mimic those from new physics signal events in FASER.
Additionally, cosmic rays may pass through the FASER detector. However, any potential background induced by them can be suppressed to negligible levels by requiring that they have high energy and point back to the ATLAS IP within the angular resolution of the detector.
Radiation level at the FASER location have also been estimated in simulations performed by the CERN EN-STI group, as well as independently measured by BatMon battery-operated radiation-monitoring devices installed at the detector location. The radiation level has been found to be low enough to allow non-radiation-hard electronics to be used in the detector.
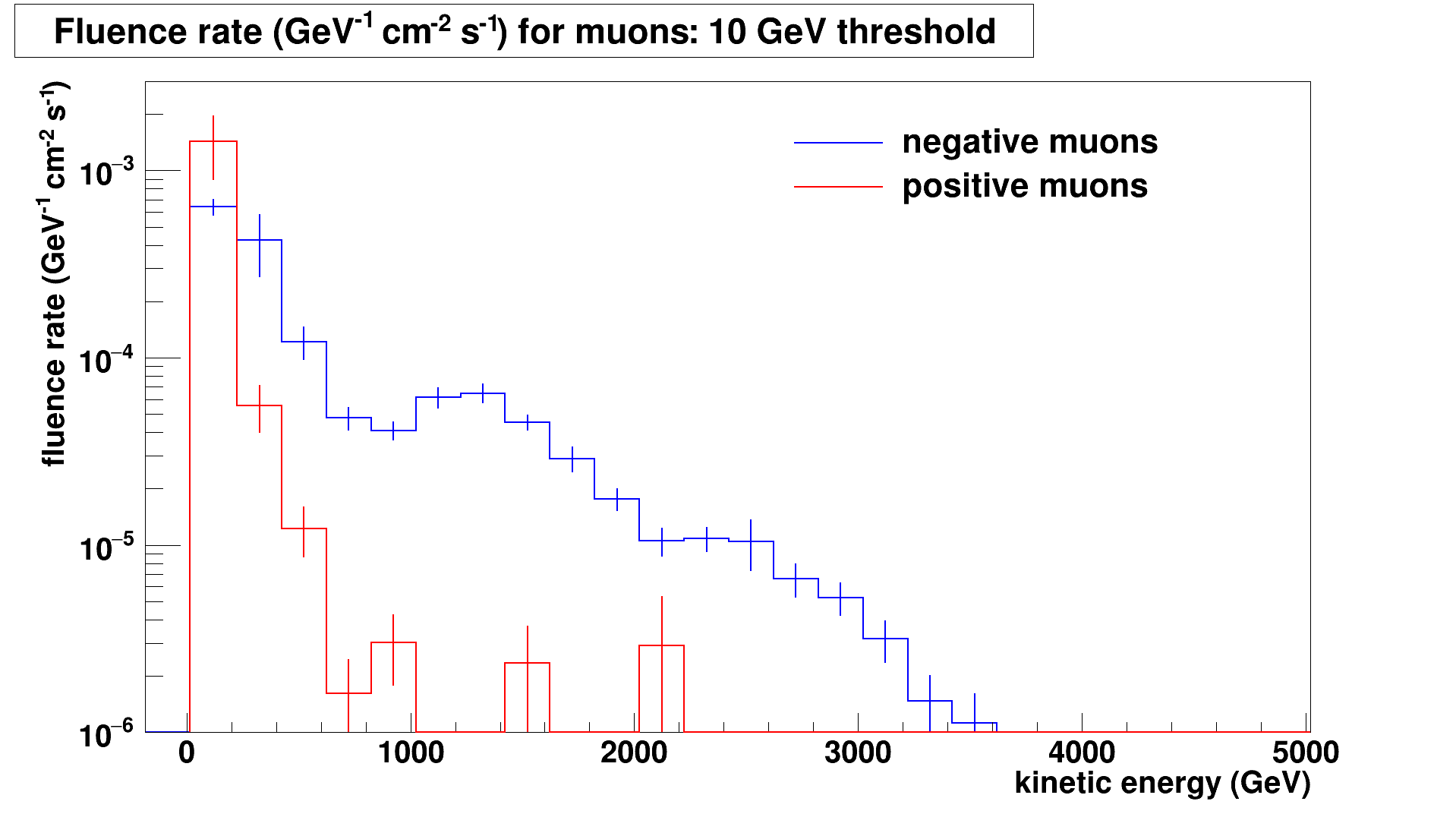
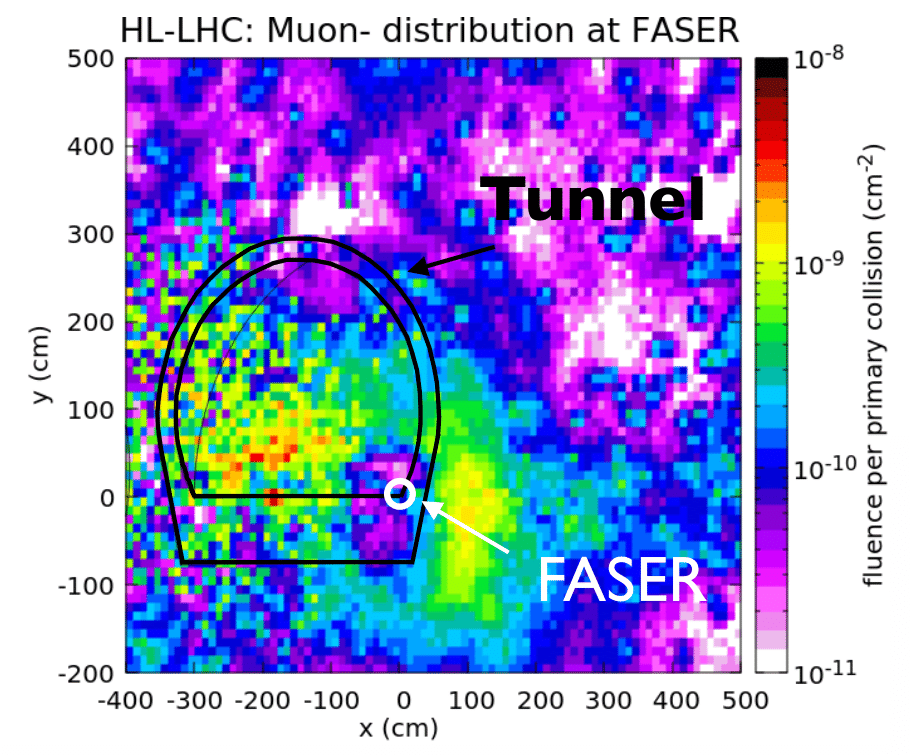
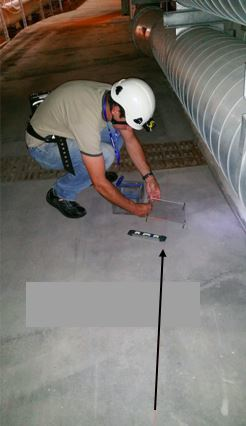
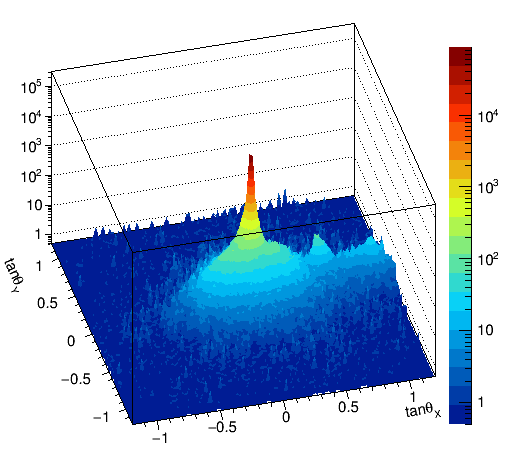